Experimental Biophysics of Complex Systems
- About research group
- Members
- Equipment
Head: Professor Štrancar Janez, PhD
Code: P1-0060
Duration:January 1, 2019-December 31, 2024
January 1, 2015–December 31, 2018, head: Professor Štrancar Janez, PhD
January 1, 2009-December 31, 2014, head: Professor Serša Igor, PhD
January 1, 2004-December 31, 2008, head: Professor Štrancar Janez, PhD
International collaboration: Elettra Sinhrotron Trst (I)
Institut for biophysics and nanosystems research (A)
University of Wageningnu (NL)
Mayo Clinic, Rochester MN, (ZDA)
The University of Utah, Salt Lake City UT, (ZDA)
Program group Experimental biophysics of complex systems and imaging in biomedicine combines research of processes and structures of biological systems by developing new advanced experimental techniques of super-resolution microscopies, microspectroscopies and nanoscopies as well as new imaging techniques. Our research is mainly focused on the response of molecular and supramolecular structures to interactions between materials and living cells as well as between light and living cells. We are interested in molecular events and physical mechanisms with which these events are causally connected, time scales, conditions and applied value of the investigated mechanisms, especially for use in medicine and in the field of health care in general. With the development of new coupled super-resolution and spectroscopic techniques we want to open new possibilities to investigate biological systems and from there onwards to open new possibilities for designing medical materials and devices, for diagnostics, therapy and tissue regeneration, representing key challenges due to the population aging. The investment into the new super-resolution STED system opened variety of fluorescence microscopy approaches: STED microscopy and two-photon (2PE) microscopy, multichannel spectrally resolved fluorescence lifetime imaging (spFLIM), fluorescence microspectroscopy (FMS). These, coupled with optical tweezers, can be used to examine interactions between materials, nanomaterials and cell lines and the phenomena involved such as lipid wrapping, membrane disintegration, and cellular membrane translocation bypassing conventional signaling pathways. We also introduced a method that enables monitoring of the electric field in tumors in the treatment of cancer with electroporation, and further developed a method of multiparametric magnetic resonance imaging for the characterization of food and medicines and various industrial processes. High resolution magnetic resonance imaging can monitor the effectiveness of surface treatments, the formation and dissolution of gels as well as measure diffusion in confined geometries with the use of modulated gradients.
Because of the introduction of nonlinear super-resolution live microscopy in 2017 to monitor changes in supramolecular structures in living systems and deep involvement in H2020 SmartNanoTox project, in 2018 the team of Laboratory of Biophysics successfully published its first high-impact paper after many years. In this publication (Nano Lett., 2018, 18 (8), pp 5294–5305) we directly observed a molecular event of lipid wrapping in the lung epithelium by STED microscopy, which we could previously observe only indirectly, using many other methods. This observation has triggered many other studies, including proteomics analysis, which has been done within SmartNanoTox consortia, in particular with David Gomez group at UCD (Dublin, Ireland) and which indicated many possible interferences between various proteins, their relocation and the known signaling cascades. The paper published in Nano letters has discussed one of those interferences, the one that potentially lead to coagulation. However, the proteomics results led us to lysosome disruption, mitochondrial network changes, and cytoskeleton degradation as well, which have been studied by live STED microscopy in 2018. Several interesting phenomena has been observed driven by physical affinity between surface of nanomaterials and supramolecular structures such as fibrous structures of actin and tubulin and membranes of mitochondria, endosomes and lysosomes. The team members have focus on the dynamics, the driving forces and correlation by the known processes such as endocytosis, linear transportation within the cell and uptake into lysosomes where the cell would try to degrade nanoparticles, of course unsuccessfully. As a results of such physical interactions, new structures have been observed making a complex of remnants of cell organelles and nanomaterial. More evidences are collected to prove that these structures are growing within the cells, before the cells go into apoptotic phase.
To understand the first contact between nanomaterials and lung epithelial surface, the Laboratory of Biophysics team has started a development of a living copy of a lung together with a system that mimics breathing including nanomaterial exposure. Here the problem addressed in our lab is the rupture of the surfactant layers that separates the air in the lung and the epithelial cell layer. Nebulization of nanomaterials has been implemented on special incubator that allows monitoring of the cell layer by STED super-resolved microscopy in live during exposure. Since nanomaterial exists as individual particles as well as various aggregates, high dynamic range vertical-section microscopy has to be applied free of PSF contamination. For that purpose, the other modality of new equipment two-photon STED microscopy has been applied (at the time of purchase this was the only commercial machine of this kind in the world) and allow us to see “nano rain” in vertical cross-section. To colocalize with surfactant proteins SP-B and SP-C the team has get in contact with the group of Jesus Perez Gil from Univ. Madrid (Spain), which will provide us specific labeling of the aforementioned proteins.
To track nanoparticles in a living organism, NP must be have fluorescence signal. In the past year Laboratory of Biophysics team has designed and optimized NP labeling protocol that is suitable for metal oxides in general, exemplified on TiO2 NP. This protocol contains an important step, for which the basic knowhow has been transferred into our lab from group of Christian Eggeling from Univ.Oxford (UK). This step relies on the Fluorescence Correlation Spectroscopy that can be performed also on our STED microscope. FCS is used to validate the labeling efficiency and desorption of the probe after labelling. In addition to the labelling protocol, our team has discovered that (at least some of) the TiO2 nanostructures can be imaged without labelling by gated microscopy. The trick originates in the fact, that some of TiO2 structures weakly fluoresce. Usually, this fluorescence is so fast that normal detectors do not acquire enough light. In our case, APD detectors are fast enough, providing an opportunity to catch those photons fast enough and distinguish them from other fluorescence such as autofluorescence or the one from specific labeling. This result has been recognized by European Commission and the SmartNanoTox officer at SmartNanoTox midterm evaluation in September 2018 as one of the most important results in nanotoxicology-related EU projects since it allows tracking of TiO2 nanomaterials in vitro, in vivo and ex vivo without labelling.
For efficient labelling we have designed, synthesized and tested a series of new STEDable fluorophores where we specifically aim at molecules that rearrange slowly from plasma to internal cell membranes. Two candidates have been selected for further improvements based on the stability/possible resolution and slow rate of relocation in lipid phase. In addition, we designed new par-fluorophores for nano-temperature mapping, which aim at detecting temperature profiles within cells, in particular within mitochondria, where higher temperature are expected based on evolution adaptation of mitochondria-located enzymes.
In collaboration with Biological Physics Group at Carnegie Mellon University, Pennsylvania, US, we have studied phase behavior and structure of lipid sphingomyelin (SM) model membranes using X-ray diffraction. Despite the biological significance of SMs, there is far less structural information available for SMs compared to glycerophospholipids. We have observed clear evidence of a ripple phase for egg SM as well as palmitoyl SM for a relatively broad temperature range below the main phase transition temperature. This contradicts the usual assumption that SM mebranes are in the gel phase below the main phase transition. Therefore, these findings suggest necessity to re-evaluate interpretations of structural results obtained on physiologically relevant model lipid rafts, which contain SM as one of the lipid components. In addition, the obtained electron density profiles in the fluid phase will allow development of improved force fields for molecular dynamics simulations.
In collaboration with University of Oxford (UK) we showed that activation of T cells during microscopy can only be prevented by suspending them in a hydrogel. The findings of the study involving superresolution STED microscopy were published in Nature Immunology. Using super-resolution fluorescence correlation spectroscopy (STED-FCS) we showed that slow diffusion of proteins in the lipid envelopes of proteins is mainly due to their composition, and not their curvature.
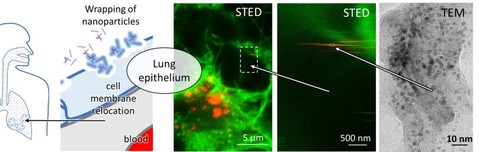
Use of magnetic resonance in wood science
Magnetic resonance imaging is a very efficient method for water detection in biological systems, which certainly includes wood. Water has a major influence on wood properties, especially dynamics moisture cycles, which affect wood in outdoor applications. It is therefore important to understand the penetration and distribution of water in the wood. In collaboration with colleagues from the Department of Biotechnical Faculty, University of Ljubljana, we conducted a study in which a rainfall events were simulated to correspond to water immersion periods of one hour. For the study the most important wood species in Central Europe with different water repellents properties were used. After the immersion, the samples were imaged by MRI. Measurements were used to determine the water distribution in the wood and to elucidate changes during the drying of the specimens. From these measurements a scientific article was published: Mojca Žlahtič Zupanc, Urša Mikac, Igor Serša, Maks Merela, Miha Humar. Water distribution in wood after short term wetting. Cellulose, ISSN 0969-0239, First Online 09 November 2018, https://doi.org/10.1007/s10570-018-2102-y.
Use of magnetic resonance in battery research
Due to the increasing number of mobile devices, the development of more powerful batteries is necessary. MRI can be used to monitor the growth of dendrites in batteries, and by using a special MRI technique, CD-MRI, also to monitor the electric current during battery operation. However, batteries contain metal parts (electrodes) that cause distortion in the MR image. Therefore, the influence of metal electrodes on the MRI signal in different orientations with respect to static and radiofrequency magnetic fields was tested on a model battery, and the optimal orientation of the electrodes in which the distortions are practically negligible was determined. The results were published in the article: Igor Igor, Mikac Urka. A study of MR signal reception from a model for a battery cell. Journal of magnetic resonance, ISSN 1090-7807, 2018, 294, p. 7-15.
Deformation tensor imaging by magnetic resonance
Magnetic resonance imaging enables precise detection of phase changes. This among other effects enables also the measurement of very small displacements in the direction of the applied magnetic field gradient. In the magnetic resonance imaging laboratory, we have shown that by the use of the spin-echo imaging method with a superimposed bipolar gradient pair (the PGSE method) displacements can be measured with accuracy of up to 0.7 μm. With experiments of the test sample, we showed that this method enables also imaging of deformation tensor. These results were published in the article Serša Igor. Magnetic resonance imaging of strain in elastic gels. Journal of Applied Physics, ISSN 0021-8979, 2019, 125(8), p. 0825211–082521-9.
Simulations of translational dynamics of chain-like particles through mucosal scaffolds
Mucus scaffolds represent one of the most common barriers in targeted drug delivery and can remarkably reduce the outcome of pharmacological therapies. Understanding the transport mechanism is particularly important for treatment of disorders such as cystic fibrosis. In the study, we employed the bond fluctuation model (BFM) to analyze the effect of steric interactions on slowing the translational dynamics of compound chain-like particles, traversing through scaffolds of different configurations. The developed mathematical model accounted for the geometry-imposed steric interactions as well as for the intra-chain steric interactions between the chain subunits. The presented model is generic and could be applied also for studying the translational dynamics of other particles with more complex architecture, such as dendrites or chain-decorated nanoparticles. The results of the study were published in Bajd Franci, Serša Igor. A bond-fluctuation model of translational dynamics of chain-like particles through mucosal scaffolds. Biophysical journal, ISSN 0006-3495, 2018, 114(11), p. 2732-2742.
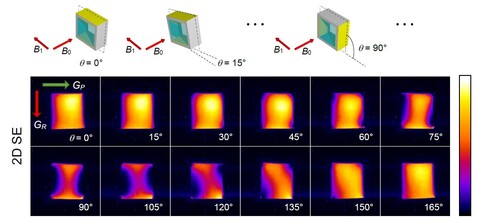
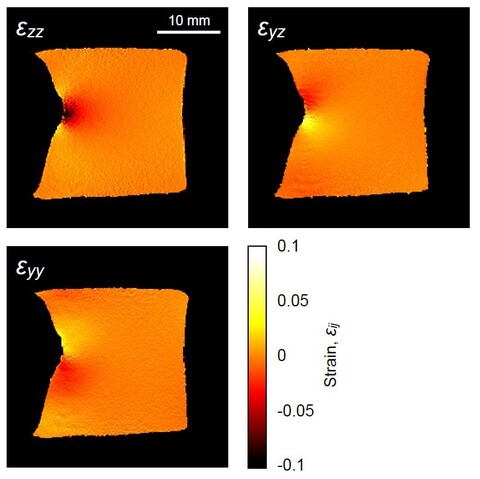
Name and Surname | Role | Laboratory | Room number |
---|---|---|---|
Janez Štrancar | Head of the research group | LBF | J109 |
Tilen Koklič | Researcher | LBF | |
Boštjan Kokot | Researcher | LBF | |
Hana Kokot | Researcher | LBF | |
Benjamin Koroševič Koser | Young Researcher | LBF | |
Mojca Urška Mikac | Researcher | MRI | J418A, 009A |
Aleš Mohorič | Researcher | ||
Rok Peklar | Young Researcher | MRI | |
Ana Sepe | Technician | MRI | 009A, C304 |
Igor Serša | Head of laboratory | MRI | J418A, 009A |
Cody Tripp | Technician | LBF | |
Iztok Urbančič | Researcher | LBF | J107 |
Laboratory for cultivation of bacteria
Equipment: C2 laminar flow cabinet, incubator, shaker, fume hood, spectrophotometer, centrifuge, Nikon fluorescent microscope with cell-counting software, different lenses and computer-controlled stage
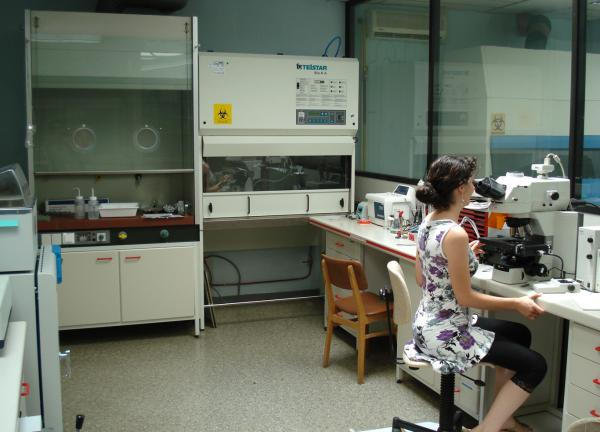
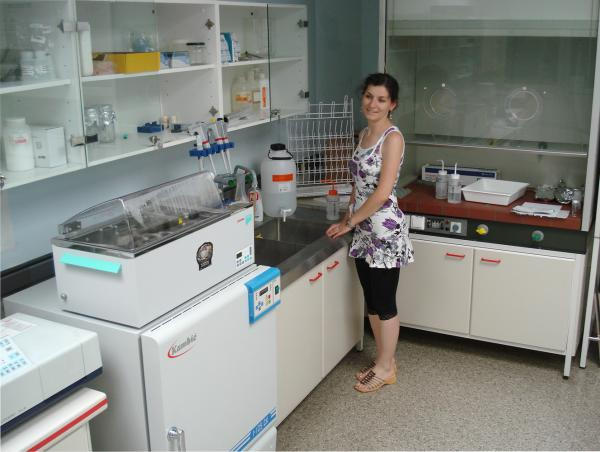
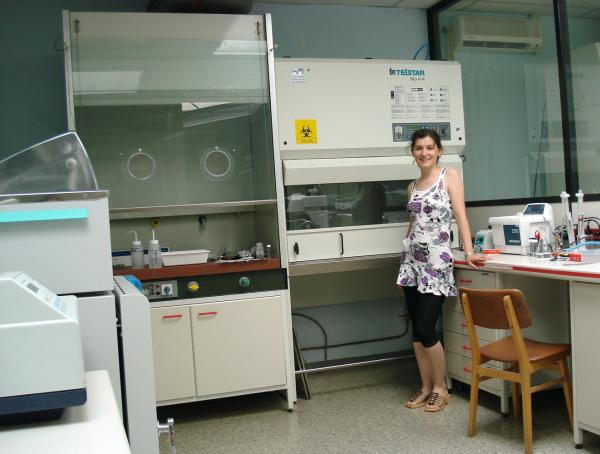
Laboratory for cultivation of eukaryotic cells
Equipment: laminar flow cabinet, incubator, ultracentrifuge, -70°C refrigerator, other minor equipment
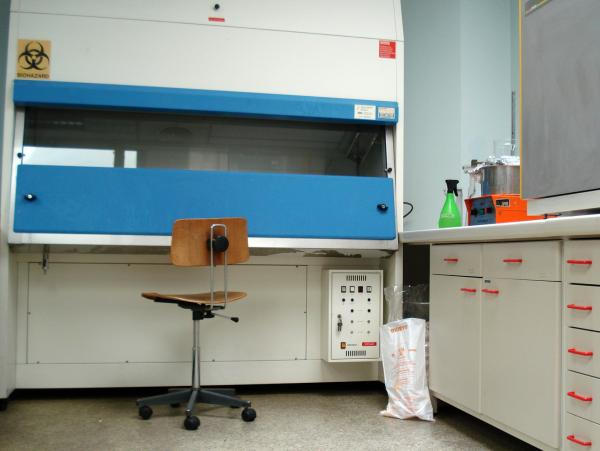
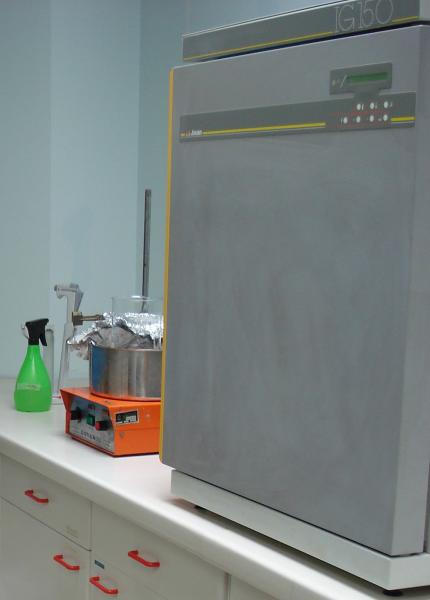
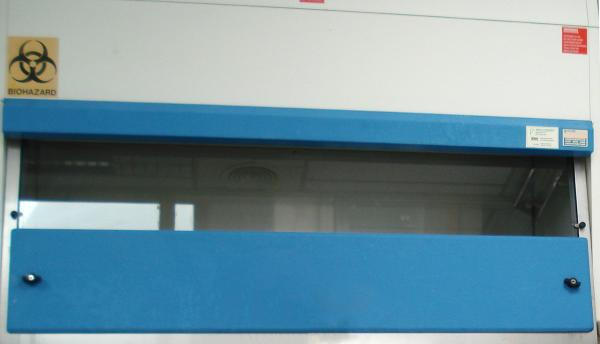
Buchi R-200 rotary evaporator
Rotary evaporator for preparation of spin films and suspension membranes (liposomes) powered by a water pump with automatic control and pressure regulation
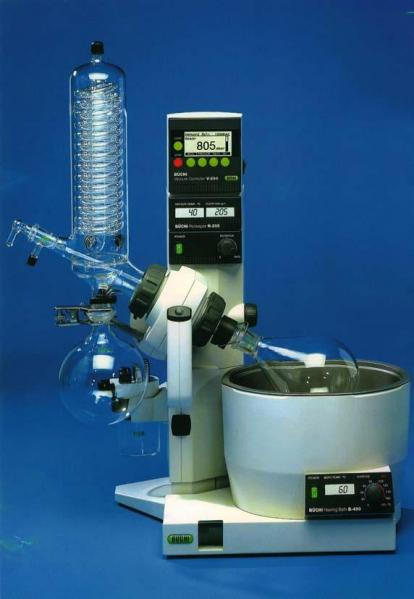
Philips 301 transmission electron microscope (worn-out)